CMS measures the W boson mass with record precision
The mass of the W boson, a cornerstone of the Standard Model, is the focus of increasingly precise measurements aimed at testing the foundations of particle physics. Following the publication by the CDF collaboration of a value that diverged from theoretical predictions and previous results from experiments at LEP and the LHC, the CMS collaboration has now produced a new measurement—the most precise ever conducted at the LHC—which aligns with the model's predictions.
The W boson, together with the Z boson, mediates the weak interaction and plays a central role in the Standard Model. Its mass, predicted by the theory based on parameters such as the Higgs boson and top quark masses, is 80,355 ± 6 MeV. These three masses are interconnected in the Standard Model, meaning that improved precision in their experimental measurements could uncover discrepancies—potential signs of new physics.
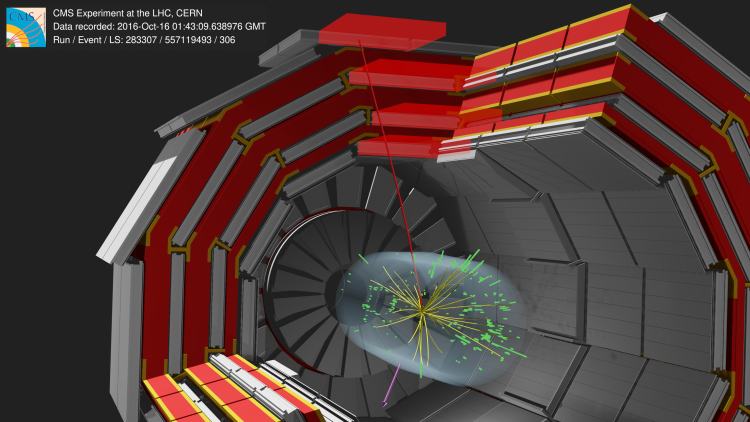
After analysing a portion of the data collected at the LHC in 2016—around 100 million events involving W bosons, the largest sample ever used for this type of measurement—the CMS collaboration announced in September 2024 a new result: 𝑚𝑊 = 80,360.2 ± 9.9 MeV. This represents the most precise measurement of the W boson mass to date at the LHC. In excellent agreement with theoretical predictions, this result reinforces the foundations of the Standard Model, despite the notable discrepancy with the value reported by the CDF collaboration in 2022. CDF had measured a value inconsistent with both theoretical expectations and results from other experiments, including those at LEP, the Tevatron, and the ATLAS and LHCb collaborations (see box).
A major experimental challenge
While W bosons are produced in abundance at the LHC, measuring their mass is a major experimental challenge. When they are produced at the centre of the CMS detector during a proton-proton collision at the LHC, they decay almost instantaneously into other particles. The mass of the W boson cannot be measured directly; instead, it is inferred through detailed analysis of its decay products. CMS physicists focused on decays of the W boson that produce a muon and a neutrino. Since neutrinos escape detection, the measurement relies on the precise determination of the muon's momentum. The CMS result is built on an exceptionally accurate measurement of the transverse momentum and pseudorapidity distributions, achieved through advanced muon reconstruction and calibration techniques, combined with cutting-edge theoretical predictions.
One of the primary challenges in this analysis was ensuring that the modelling of the data was accurate and that the extracted value of the W boson mass was unbiased. To address this, CMS turned to another particle, the Z boson, whose mass is well-established and which decays into two muons that are clearly detected by CMS. By ignoring one of the two muons—treating it as if it were a neutrino—they were able to measure the Z boson mass using the same method applied to the W boson. This was achieved by calibrating the muon momentum without relying on the Z boson mass. Remarkably, the tests showed consistent results, effectively ruling out any experimental biases in the measurement.
In France, members of the CMS collaboration at the Laboratoire Leprince-Ringuet (LLR, CNRS, École Polytechnique) played an important role in this sophisticated analysis, contributing both to its development and to the revision process over several years. Andrew Gilbert provided critical leadership as coordinator of the Standard Model Measurements group over the past two years, guiding the revision process. Additionally, Roberto Salerno, currently CMS Physics Coordinator, continues to oversee and support this work.
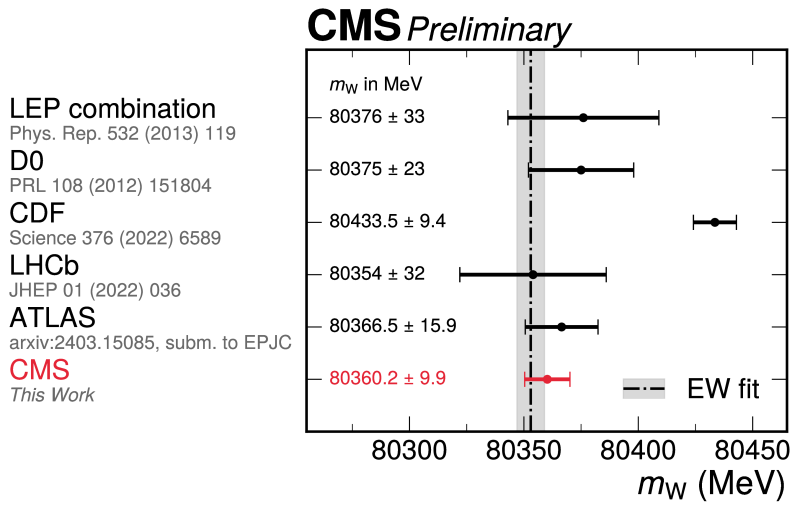
A Precision Journey
Since its discovery at CERN in 1983, the W boson mass has been a key focus in particle physics. The LEP experiments at CERN established the first precision benchmark with a combined accuracy of 33 MeV. Later, the D0 and CDF collaborations at the Tevatron achieved even greater precision. With the start of the LHC era, landmark results followed, including those announced by ATLAS in 2016 and LHCb in 2021, both of which aligned with the predictions of the Standard Model.
However, in 2022, the Tevatron CDF collaboration published a surprising measurement of 80,433.5 MeV, with an uncertainty of just 9.4 MeV. This value diverged significantly from theoretical predictions, igniting an animated debate within the scientific community. In 2023, the ATLAS collaboration presented an updated measurement (80,366.5 MeV with an uncertainty of 15.9 MeV) from a new analysis of LHC Run 1 data. This value was consistent with previous measurements, with the exception of the CDF result. In this context, the new CMS measurement makes a decisive contribution with a precision comparable to that of CDF and confirms the prediction of the Standard Model.